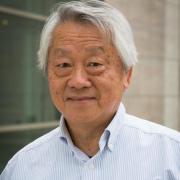
Yuh Nung Jan, PhD
Neuronal morphogenesis and circuit assembly
We are interested in the basic mechanisms of neural development. Our strategy is to use the relatively simple Drosophila peripheral nervous system (PNS) to discover the genetic program that controls its development. In doing so, we hope to uncover evolutionarily conserved core programs that control different steps of neural development in animals. We started with the earliest steps in neural development (neurogenesis and neuronal cell fate specification) and gradually worked our way toward later steps (neuronal morphogenesis and the assembly of a functional neuronal circuit). Some highlights of our earlier efforts include the discoveries of atonal and numb. Atonal is a basic-helix-loop-helix (bHLH) protein, which is the founding member of an important family of proneural genes that function to initiate the development of two major types of sensory neurons used in vision and hearing. Its mammalian homologues include Neurogenin, Math 1 and Math 5. numb functions as a cell fate determinant during asymmetric cell division. Numb provided a starting point for the study of asymmetric cell division in Drosophila and vertebrates and led to considerable insight into the molecular basis of asymmetric cell division. In recent years, the major focus of our lab has shifted to the study of dendrite development and neuronal circuit assembly.
Current Projects
(A) Mechanisms controlling dendrite development in Drosophila.
Dendrite arborization patterns are critical determinants of neural circuit formation and influence the type of synaptic or sensory inputs a neuron is able to receive. Moreover, dendrite defects are associated with a variety of human mental disorders such as autism. Relatively little is known about the molecular mechanisms that control dendrite development. We use the fly transgenic technique to express green fluorescent protein (GFP) in the dendritic arborization (da) neurons, a group of sensory neurons with a stereotyped dendritic branching pattern. This allows us to visualize the development of the dendrites of da neurons in the living fly embryos and to use them as an assay system for a genetic dissection of neuronal polarity and dendrite development to gain insights about the mechanisms underlying (1) how axons and dendrites are made differently, (2) how a neuron acquires its neuronal type specific morphology, (3) how the dendrites of different neurons are organized, (4) how the size of a dendritic arbor is controlled, and (5) how the pruning and remodeling of dendrites are regulated during development.
(1) Dendrite specific developmental regulators. Similar to the majority of vertebrate neurons, the Drosophila da neurons show clear dendrite vs. axon polarity including the orientations of microtubules (MT). It is well known from numerous studies in various organisms that MT cytoskeleton plays a major role for the proper establishment and maintenance of neuronal architecture. For example, the differences in MT polarity in dendrites and axons have substantial impact on the structural and functional differences of axons and dendrites. An important unanswered question is how MTs are nucleated in neurons. Many recent studies point to the existence of acentrosomal MT nucleating sites. We recently found that Golgi outposts serve this role in the dendrites of Drosophila da neurons.
From our mutant screen, we identified a group of dar (dendritic arborization reduction) genes. Mutations of any of the dar genes lead to defective dendritic arbor but normal axonal projections. Thus, studies of dar genes should reveal how axons and dendrites are made differently. We estimate that there may be a total of about 20 dar genes in Drosophila. Of the 5 dar genes that we have cloned so far, all five have mammalian homologues. Remarkably, three encode components of the secretory pathway. These results reveal the preferential role of ER-Golgi trafficking and Golgi outposts in dendrite arborization.
(2) Transcription factors regulate dendritic field size and complexity. Transcription factors are important regulators of the size and complexity of dendritic fields and the logic of their usage is beginning to emerge. For example, in the Drosophila PNS, the Zn-finger containing protein Hamlet functions as a binary switch between the elaborate multiple dendrite morphology of da neurons and the single, un-branched dendrite morphology of external sensory (es) neurons. In most cases, however, the dendritic morphology would be determined by the combined action of multiple transcription factors such as Cut and Spineless.
(3) The molecular mechanism for dendritic self-avoidance and tiling. Dendrite-dendrite repulsion can have a profound influence on determining the size and shape of the dendritic field as well as the spatial relationship between different dendritic fields. The dendrites of each da neuron show self-avoidance and tend to spread out without crossing over. Class III and Class IV da neurons show tiling, i.e., there is very little overlap between the dendritic fields of adjacent neurons of the same class because their dendrites show homo-typic repulsion. We found that Dscam, originally identified as axon guidance receptors by Larry Zipursky and his colleagues, are needed for self-avoidance and contribute to the spreading of dendrites. Without Dscam, the dendrites of each da neuron would bundle together or cross over. Parallel and complementary works were done in the labs of Wes Grueber, Larry Zipursky and Dietmar Schmucker. In contrast, tiling requires some cell surface recognition molecules other than Dscam to mediate the homo-typic repulsion. Although the signal(s) that mediate tiling behavior remain elusive, we recently discovered that integrins regulate repulsion-mediated dendritic patterning of sensory neurons by restricting dendrites to a 2D space as defined by the extracellular matrix (ECM). The previously identified tiling mutants of the Tricornered (Trc)/Furry pathway affect dendritic tethering to the ECM and thus allow the dendrites to cross over in different planes.
(4) The maintenance of dendritic fields. Our genetic screen revealed specific mechanisms that function to ensure maintenance of dendritic arbors. We found that components of the Hippo pathway including the tumor suppressor Warts (Wts) as well as the Polycomb group of genes are required for the maintenance of the class IV da dendrites. Loss-of-function mutations of any of those genes cause a progressive defect in the maintenance of dendritic tiling, resulting in large gaps in the receptive field. We are continuing our study of how the establishment and maintenance of dendritic fields are coordinated.
(5) The remodeling of dendritic fields. Drosophila class IV da neurons undergo dramatic remodeling during metamorphosis. Early in the pupal stage, those neurons prune all their dendrites. Later they grow a completely new dendrite for adult function. While the dendrites are being remodeled, the axons stay largely intact. We have continued to identify the molecular mechanisms that control this large scale dendrite specific remodeling.
(B) Functional implication of dendrite morphogenesis and the study of mechanosensation.
To understand dendrite morphogenesis, we felt that we need to study it in the context of function. The fly uses a variety of mechanisms such as self-avoidance and tiling in order to form a beautiful array of class IV da neuronal dendrites to tile the body wall. Why? Based on the work of several labs including ours, we know that class IV da neurons are poly-model nociception receptors: they can sense high temperature or noxious mechanical stimuli. Recently, we discovered that class IV da neurons also function as photoreceptors. It turns out that Drosophila larvae have two different types of photo-sensors. The previously known larval photoreceptors, the Bolwig organ, function primarily in avoidance of low light, whereas class IV da neurons constitute a second photoreceptor system using a novel photo-transduction machinery to sense harmful short wave length light. This regular array of photo-sensors enable the larvae to sense light exposure over its entire body to move out of danger. This may explain why the organization of class IV da neurons is so similar to that of tiled neurons in the vertebrate retina.
We have also begun to use da neurons to study mechanosensation. Mechanosensation is a very interesting and understudied research area. Among our senses, mechanosensation is the least well understood as compared to vision, olfaction and taste. Part of the reason that little is known about the molecular mechanisms underlying mechanosensation is the difficulties in identifying mechanical transducing molecules. Drosophila PNS has emerged as an excellent system to study mechanosensation. At least three of the 4 classes of da neurons are involved in different modes of mechanosensation. Recently, Ardem Patapoutian’s lab discovered that Drosophila Piezo is a mechano-sensitive channel that mediates mechanical nociception by class IV da neuron, whereas our lab found that NompC is a mechano-sensitive channel that mediates gentle touch by class III da neurons and sound sensing by chordotonal neurons. Drosophila PNS promises to provide important insights about mechanosensation.
(C) Degeneration and regeneration of dendrites and axons.
Recently, we found that after severing, the class IV da neuron’s axon regenerates in a manner strikingly similar to that of mammalian dorsal root ganglion neurons after injury, namely it regenerates well in the periphery but poorly in the CNS. Furthermore, as in mammals, activating the Akt pathway enhances axon regeneration of da neurons in the Drosophila CNS. Those results strongly suggest that class IV da neurons can serve as an excellent model system for uncovering and studying the evolutionarily conserved axon regeneration mechanisms. We are currently performing a candidate-based screen, as well as unbiased screen to identify potential inhibitors for CNS axon regeneration. This strategy offers an opportunity to gain insights into the repertoire of regulators for axon regeneration, which may ultimately inspire novel treatments for nerve injury and neurodegenerative diseases. Further, class IV da neurons are not only capable of axon regeneration but also display dendrite regeneration. We have begun to compare the mechanisms for dendrite versus axon regeneration, an interesting subject that has hardly been studied in any system.
(D) Extension of our Drosophila work to dendrite development of mammalian central neurons and potential implications in neurological disorders.
We have been using the knowledge gained from our Drosophila studies of dendrite development and trying to extend that to mammalian cortical neurons. Our previous work suggests that the Hippo kinase signaling pathway has a central role in controlling dendrite morphogenesis in Drosophila. Recently, we demonstrated that NDR kinases, the mammalian homologues of Trc kinase in the Hippo pathway of Drosophila, have an evolutionarily conserved role in controlling mammalian dendrite morphogenesis. Furthermore, in collaboration with Dr. Kevan Shokat at UCSF, we have succeeded in applying the chemical genetic approach his lab has developed to identify substrates of NDR kinases, which turned out to have important functions in dendrite morphogenesis. We have continued to apply this powerful technique to identify the substrates of the mammalian homologues of other important kinases in the Hippo pathway and to study their roles in dendrite morphogenesis. Some of the substrates have been implicated in certain neurological disorders such as autism and schizophrenia.
(E) Drosophila behavior and underlying neuronal circuitry.
Our lab has also contributed in characterizing certain adult behaviors in Drosophila, including egg-laying site selection, female post-mating switch from receptive behavior to rejection of male courtship, rival induced prolongation of mating duration in the male, and social influence of male aggression. We have made progress in identifying components of neuronal circuitry underlying those behaviors.
Lab Members
General Staff
Monika Avdeef
Administrative Assistant
[email protected]
Sandra Barbel
Lab Manager
[email protected]
Tong Cheng
Lab Tech
[email protected]
Cecilia Concengco
Lab Assistant
[email protected]
Rose Lau
Adminstrative Assistant
[email protected]
Yuk Lan Seto
Lab Assistant
[email protected]
Eirish Sison
Lab Tech
[email protected]
Function Group
Chao Chen
Postdoctoral Fellow
[email protected]
David Crottes
Postdoctoral Fellow
[email protected]
Tina Han
Postdoctoral Fellow
[email protected]
Mu He
Postdoctoral Fellow
[email protected]
Sarah Headland
Postdoctoral Fellow
[email protected]
Cindy (Kexin) Li
Postdoctoral Fellow
[email protected]
Yuan-Hung Lin
Neuroscience Graduate Student
[email protected]
Christian Peters
Postdoctoral Fellow
[email protected]
Chin Fen Teo
Postdoctoral Fellow
[email protected]
Jason Tien
Postdoctoral Fellow
[email protected]
Wenlei Ye
Postdoctoral Fellow
[email protected]
Wei Zhou
Postdoctoral Fellow
[email protected]
Mario Zubia
Graduate Student
[email protected]
Development Group
Laura Devault
Neuroscience Graduate Student
[email protected]
Yanmeng Guo
Postdoctoral Fellow
[email protected]
Jacob Jaszczak
Postdoctoral Fellow
[email protected]
Peng Jin
Postdoctoral Fellow
[email protected]
Matthew Klassen
Postdoctoral Fellow
[email protected]
Tun Li
Postdoctoral Fellow
[email protected]
Wenyu Luo
Postdoctoral Fellow
[email protected]
Shan Meltzer
Neuroscience Graduate Student
[email protected]
Caitlin O'Brien
Postdoctoral Fellow
[email protected]
Maja Petkovic
Postdoctoral Fellow
[email protected]
Beverly Piggott
Postdoctoral Fellow
[email protected]
Katherine Thompson-Peer
Postdoctoral Fellow
[email protected]
Smita Yadav
Postdoctoral Fellow
[email protected]
Susan Younger
Research Specialist
[email protected]
Wei Zhang
Postdoctoral Fellow
[email protected]