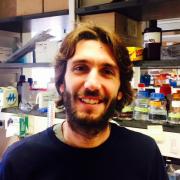
Daniele Canzio, PhD
The role of cell surface diversity in the assembly of neural circuits
The appropriate transmission of signals and effective processing of sensory and motor information demands the wiring of neurons into functional circuits. Such a process is dependent, in part, upon the ability of neurites – axons and dendrites – to project into distinct regions of the nervous system to form selective neuronal connections. Essential to neuronal connectivity is the ability of sister neurites (neurites from the same neuron) to distinguish self from non-self to avoid clumping or synaptic engagement. This mechanism is known as self-avoidance and requires that, in principle, every neuron must express a unique combination of cell surface recognition molecules to generate a molecular recognition code, i.e. an identity. Given that the human brain is composed of over 100 billion neurons, the process of self-avoidance entails the need of generating an enormous diversity of molecular barcodes. The long-term goal of our laboratory is to dissect the molecular mechanisms behind the generation of such self-recognition code in vertebrates.
Clustered Protocadherins genes: a code for neural cell surface diversity
In vertebrates, the regulation of self-avoidance has been primarily attributed to the clustered Protocadherin (Pcdh) proteins. The extraordinary functional diversity of Pcdhs reflects the unique genomic arrangement of the Pcdh genes into three closely linked clusters (a, b, g), and the elegant mechanisms for generating protein isoform diversity. These mechanisms involve (1) stochastic promoter activation of random sets of Pcdh a, b and g isoforms by a coupled mechanism of long non-coding RNA (lncRNA) transcription and DNA de-methylation, and (2) an unusual transcription and processing of Pcdh transcripts with pairing of 5’ and 3’ splice sites that are encoded in the genome at distances as great as 300,000 base pairs (bp). Therefore, the generation of a Pcdh-based diversity code requires functional coupling between distinct processes of eukaryotic gene expression such as chromosome organization, chromatin architecture, transcription and RNA processing.
Our goal is to study both transcriptional and post-transcriptional mechanisms involved in the generation of a Pcdh diversity code. Specifically, we aim to ask:
1. What is the role of long non-coding RNA (lncRNA) transcription in promoting stochastic Pcdh promoter choice?
2. What is the role of DNA de-methylation by Tet proteins in promoting random Pcdh promoter choice?
3. How are lncRNA transcription and DNA de-methylation coupled?
4. What is the mechanism by which CTCF and Cohesin promote and maintain the choice of an active Pcdh promoter?
5. Pcdh pre-mRNAs can be as long as 300,000 bp. How are they transcribed by RNAPII?
6. Correct processing of a Pcdh pre-mRNA requires pairing of 5’ and 3’ splice sites that can be 300,000 bp apart. How are these Pcdh pre-mRNAs correctly spliced by the Spliceosome?
7. What is the role of the chromosome organization in regulating transcription and splicing of Pcdh pre-mRNAs?
Despite the central role of Pcdhs in the development and assembly of neuronal circuits in the mammalian brain, the molecular mechanisms by which these genes are randomly selected, transcribed and correctly processed remain largely unknown. Understanding these processes would not only provide fundamental insights into the mechanisms involved in generating functional diversity in the Pcdh gene cluster but would also reveal more general principles of transcription initiation, elongation, chromosome organization and RNA processing.
Lab Members
Daniele Canzio, Assistant Professor
Sandy M. Rajkumar, Research Assistant